Trikke Magic: Leveraging the Invisible
Draft: 23 November 2022
Most body-powered vehicles employ cranks, gears and chains to transfer rider generated momentum; none is as direct as Trikke locomotion. An invisible yet powerful mechanism, the Magic Lever, converts good rider technique into carving a great Trikke ride. Mathematical models of the dynamics and kinematics of a Trikke T78 Air were derived and incorporated into computer simulations. Typical Trikke responses to rider input were analyzed with the goal of improving riding techniques. Indeed, knowing how a Trikke works reveals several opportunities to leverage the magic.
Disclaimer
The information in this paper is true and complete to the best of my knowledge. All recommendations are made without guarantee on the part of the author. The author disclaims any liability in connection with the use of this information. Further, I am not affiliated, associated, authorized, endorsed by, or in any way officially connected with Trikke, or any of its subsidiaries or its affiliates. The official Trikke website can be found at http://www.trikke.com. The name “Trikke” as well as related names, marks, emblems and images are registered trademarks of Trikke.
How I Became a Daily Trikke Rider
Not a Trikke Rider (Yet)?
A Magic Lever
Magic became even more magical when I realized what the main power producing mechanism of a fitness or body-powered Trikke is. Of course, the rider supplies the power, but the mechanism has to be realized through levers, gears, pulleys and such - right? It took a good, long look at the Trikke frame and the progress I made riding it to analyze the [physics] of the ride. Four levers hide in that three-tube frame and 3 mechanisms bring it to life. That wasn't enough. Which mechanisms work best in which road conditions? A forward-feed, discrete, [calibrated] Trikke/Rider [simulation] demonstrated the typical [behaviors] needed to answer those questions and more. Over three years after my first ride, about an hour a day riding and an hour "hobbying", at last I was able to make a contribution to the Trikke Community. But I still wasn't perfectly clear about how I was generating the torque that feeds the physical mechanisms; until now [Quick Start], over 5 years from that first ride.
What is the main mechanism that drives a Trikke? Look at the illustration on the right to find out; Figure 3. It shows the workings of the most important of the 4 levers; the black dashed line. What makes this lever so magic is that it is not a "solid" lever as one might expect. It arises out of coordinated friction at each wheel contact patch on the road. The same friction forces that constrain wheels to roll forward, make them turn around a turn-center just like a lever rotates on a fulcrum (black dot at bottom). Every part of the Trikke must also rotate around the turn-center on a turn-center arc (i.e., orbit). Parts farther to the "outside" rotate faster than those closer to the fulcrum on the "inside". Parts in the same orbit or when the Trikke is not turning have the same speed.
One of the farthest parts is the front-wheel contact patch (center of black wheel in the upper left). This guide-wheel receives the action (purple ray). The farther out it sweeps, the faster it moves relative to the turn-center fulcrum. Closer in we find the center of mass of both the rider and Trikke (brown dot). They combine creating a system center of mass that constantly changes position; shifting with both the rider and the configuration of the Trikke via steering and cambering. This is the load (orange dot and ray) on the lever (black dashes); the stuff that gets pushed by the action in orbit around the fulcrum. While the load is not actually located on the lever, it acts as if it is on the lever simply because both the Trikke and rider revolve around the turn-center as a unit. The result is the same: give the guide-wheel a push, via a jetting action, and the center of mass follows it in its own orbit (brown ray).
Jetting turns the rider's angular momentum along the guide-wheel path (purple ray) into linear momentum at the system center of mass (brown ray). Like most systems, there is loss in this momentum transfer. One source of that loss is the ratio of the system center of mass turn-radius to the guide-wheel contact turn-radius. If it is less than one, the usual case, there is a proportional loss. At one, there is no loss due to this source. Greater than one, there is a proportional gain; this can only happen near the center-line when steering turns from a straight line. There is always a loss, just not due to this particular geometric factor. Can the rider move the system center of mass toward the guide-wheel orbit to take advantage of this? Not sure - but it would be difficult.
On the other hand, action at a right angle to the guide-wheel dissipates in wheel deformation, heat and other forms of friction as in Figure 4. Unless so strong that the wheel slips or structurally fails, radial momentum along the magic lever does not affect speed. In fact, action (purple double ray) along any turn-radius (brown line) at any point (brown dot) on the Trikke or rider meets the same fate, friction across the wheels, illustrated as red double rays. Therefore the rider's side-to-side momentum generally has no positive effect on speed! However, the weight shift can be a negative. But a rider can still take advantage of this fact to setup for a push by shifting position without affecting speed negatively.
It's not very complicated. It's virtually magic!
Three Ways to Tame a Trikke
"Carving vehicles" earned that epithet by similarities with skating sports. In particular the way that a ski or ice-skate blade cuts or melts into the surface to hold its edge. A Trikke wheel doesn't sink into the road, but its path-constraining effect is the same. This edge-grabbing characteristic is responsible for the "snake-like" paths etched by athletes in these sports. A Trikke is really good at it.
- Carving: [Physics] analysis shows that the rider can apply two mechanics to produce a carving response from a Trikke. Jetting directly throws the magic lever via the rider's intentional body rotation. Direct, linear pushing splits the action between turning the lever and increasing friction across the wheels when the rider intentionally throws weight rearward.
- 1) Jetting: This term comes from an old skiing technique used until the mid 1980's when ski materials improved dramatically. It involved shoving both feet forward to top small ridges or up-sloping turns. Dropping one's center of mass to power the jet made it difficult to maintain balance. Trikkes are much more stable and the jet is mainly performed one foot at a time - a "hemijet". The action is generated without moving the rider's center of mass relative to the Trikke. There is no recoil. Jetting is a rotation as illustrated in Figure 3. There is Conservation of Angular Momentum in conjuring a jet. When led by a hip twist and a delayed turn to the same side, the rider's spin momentum is first reflected then transferred to the Trikke-Rider pair as [co-rotation].
To generate jetting acceleration a rider can employ both lower body and upper body, or emphasize one over the other. Either way, the hips lead the upper rotating into the turn. As the lower body rotates the outside leg extends to throw the deck forward with the toes. The inside leg controls how quickly you shift your weight from the outside of the turn to the inside. As the shoulders rotate, the inside arm tries to keep its handlebar a constant distance from the body. The outside arm pushes its handlebar forcefully to accomplish the turn. As long as the inside handlebar is relatively stationary, the outside push feeds into the guide-wheel path as a jet, not so much as a push.
It is possible to jet backward, but steel Trikkes have a warning sticker that reads: "Do not roll backward". Presumably, the trailing arms could splay causing yoke damage.
Each of the other mechanisms in this list describe how various rider actions synergistically feed into jet rotation.
-
2) Pushing: When a Trikke is stationary and pointed forward, a straight (linear) push shoves the Trikke away from the rider. This "steps" the Trikke forward on the road, but it also moves the rider backward on the Trikke. The rider must reset his position on the Trikke to push again. Resetting means pulling forward; the Trikke "steps" backward on the road toward the rider as the rider moves forward on the Trikke. Physically, Conservation of Momentum guarantees the rider will gain no ground as long as wheel friction is really low as it is on Trikkes.
However when a Trikke turns, a linear push causes a forward step. But that action synergistically sets the rider up for a push when the handlebars are turned the other way. It seems like a push turning one way followed by a push turning the other way leads to a forward step and another forward step each cycle. While experience can dramatically reduce any need for a reset, usually any actual reset just goes unnoticed.
How does a straight push feed into jetting action? Any force applied to a turning Trikke, like the green action ray in Figure 5, is split into two right-angle parts at the guide-wheel contact point. One part is an orbital rotation that pushes forward along the guide-wheel path (green arrow) around the turn-center (black dot). The other part of the force dissipates in friction radially along the magic lever (black dashes). The orbital part feeds directly into a jet action as the purple ray.
In this case, Conservation of Angular Momentum is satisfied by the rider's recoil (thicker orange ray). Again, part of the recoil is rotation in his orbit (orange arrow) and part is radial (thin orange ray) that dissipates in friction across the wheels. The orbital part from the rider and the orbital part that is the jet are equal and opposite; they are conserved.
Strictly, a linear push requires both arms to push the handlebars away at the same rate, whether in a turn or not. In a turn, a push is mostly produced by the inside arm pushing its handlebar away form the body. The outside arm still pushes its handlebar more to complete the turn, but some of that is jetting and some is push. Never-the-less, part of a linear push is also jetting as explained above. The main difference is that a jet has no radial waste.
- 3) Castering: If you have watched any Trikke videos, you've probably heard the word "punching" to describe some ill-defined quick upper body action. Physically, a quick upper body action imparts a jetting impulse to the guide-wheel. This can mean a quick direct push or a quick turn attempting to super-power the castering mechanism. This mechanism operates because the guide-wheel is cast at an angle to vertical. Whenever the handlebars are turned, the yoke (where the 3 tubes come together) orbits the steering column creating a yoke-lever. First, the Trikke trailer is pulled slightly, then turning past straight ahead, the stem gets pushed slightly.
- Pull: When changing turn direction in a drive-cycle, the Yoke-Lever (blue line near wheel) rotates the yoke Y͆ pulling it closer to the guide-wheel contact point C͆σ as shown in Figure 6. The yoke, in turn, pulls on and rotates the Trailer-Lever (green line). This action results in the trailer and everything on it building up angular momentum (orange arrow). The amount depends on the steering angle, camber, position of the rider, quickness of the action and the location of the transom point. Build up continues as the steering angle passes the forward direction.
- Push: As steering slows reaching its limit on the other side in Figure 7, the built up angular momentum (green arrow) releases through a lever-arm L (brown) to the Trikke-Rider System in a jet action (purple ray). In turn, this jet rotation around the Turn-Lever moves the TRS center of mass. This part of castering is called "push" because the distance between the yoke and guide-wheel contact increases. Traveling the extra distance, the wheel spins faster. Opposite what happens during the pull, the yoke can drop vertically an inch and a half causing the trailer to guide-wheel contact distance to increase. This causes the larger trailer mass to push on the steering column. However, the effect on TRS locomotion is much less than one-percent.
Figure 6 - Caster-Pull action Figure 7 - Caster-Push action To Caster a Trikke, nothing special is required, it just happens every time the handlebars are turned. It is a small effect, but worth noting. It won't power your ride, but can extend coasting a little longer just by steering quickly.
Incremental Speed
You now know three mechanisms that feed the guide-wheel of your Trikke. Accelerating a Trikke using these mechanisms means building up the speed a little at a time; in increments. One drives a Trikke in cycles. Half of a cycle to the right followed by half of a cycle to the left. A cycle can be a bit shorter than a second or restfully long up to a few seconds. Seem short? You'll get there. At 11.5 mph, my cadence falls just short of 1.5 seconds per cycle; 42 cycles per minute. Initially over 12 mph, it gets closer to a second, but lengthens a little maintaining cruise.
From on board the Trikke, you create all the guide-wheel jet-acceleration each cycle in the local frame of reference. Watching from the side of the road, you can see that the guide-wheel travels faster than the rest of the Trikke, certainly faster than the local jet-speed. This is the global frame perspective. So, when the rider stops driving, the Trikke coasts to a stop in the global frame, but motion stops immediately in the local frame. Seems a bit odd, but physically, it's as if the rider in the local frame is blind to the world around the Trikke and can't see it moving by. The observer in the global frame sees everything but at different speed and position. But the rider can "feel" global accelerations locally.
This difference is important because both are right about the speed of the Trikke. The rider creates its local jet-acceleration every cycle, and its speed adds to the Trikke-Rider System's global speed. A graph from a simulated constant acceleration and alternating turn-angle configuration shows this in Figure 8. The average global speed (green graph) builds up as long as two local jet-acceleration (blue) spikes are added every drive-cycle. Each spike represents a jetting action, left then right. Note they are each the same height. So when the Trikke speeds up to its maximum or terminal velocity for the current conditions, the rider must continue to add local jet-acceleration to the guide-wheel. At that point the rider adds exactly as much local jet-speed as friction takes away in the global frame. Note the steps - horizontal lines - added to the average global speed are not evenly spaced vertically. They depend on global friction that changes with global speed. The higher steps occur where the friction is lowest. In the figure, the Trikke increases speed over 9 mph in less than 10 cycles.
As mentioned, the global speed of the Trikke is not the global speed of the guide-wheel. During the first half of the drive-cycle, the rider turns to a certain angle and keeps it there until the next half of the drive-cycle. After steering to the same angle on the other side, the rider holds it until the end of the cycle. So, each drive-cycle has a maximum angle that is held for about half of the cycle to each side. The global speed of the guide-wheel follows this turn-angle along a snaky path, but the Trikke moves forward at a slower speed and turns less. An approximate mathematical relationship for this slithery situation multiplies global guide-wheel speed by the cosine of the turn-angle minus some loss due to friction to get the global Trikke (system center of mass) speed. So, when the turn-angle is zero, straight ahead, the guide-wheel and center of mass speeds are equal and coasting to zero. When steered to a right angle to one side, no jet-speed can be added and the speed must be zero or the Trikke is skidding to a stop or worse.
Trikke Magic
Everyone likes magic especially when it works in their favor. Like most good magic, it's difficult to assess what is actually going on, even when you know the physical principles involved. This article intends to ground the information in the [Quick Start] Trikke riding guide in science. The mechanism of walking a box across a floor invokes the basic magic of riding a Trikke - jetting! Keep reading to understand how that can be.
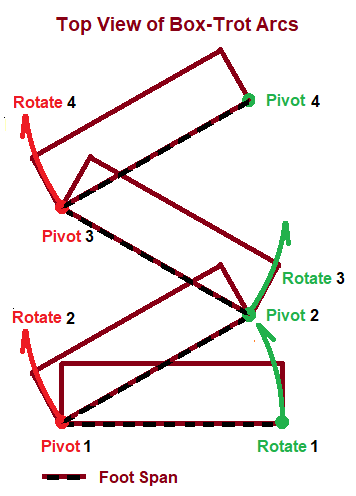
If the box is not too heavy and has the right dimensions, placing the closest edges on your toes is the easiest way to walk it. Your feet are then frozen the same distance apart and can't easily move relative to each other throughout the effort. Instead, one foot must rotate arced around the pivot provided by the other as in Figure 9. When you master this "Box-Trot", the pivot foot does not rotate at the ankle, instead it is turned as a unit by the body rotating on the ball of the foot. This motion begins at the pivot-side hip rotating the spine and upper body slightly ahead of the lower body. As the upper body gains angular momentum, the core muscle groups lock the lower body into the rotation increasing the body's angular momentum. The whole body seems to rotate together. Very difficult to see on a video. Synergy follows:
- The foot to the outside of the rotation (outside foot) raises ever so slightly with the hip twist to pass over the floor.
- Your body and the box's minimal axis of angular inertia are almost parallel and close together making it easy to turn the box with you.
- At the end of rotation, your outside foot has moved forward on an arc. Alternating pivot feet and rotations then moves you forward on arcs.
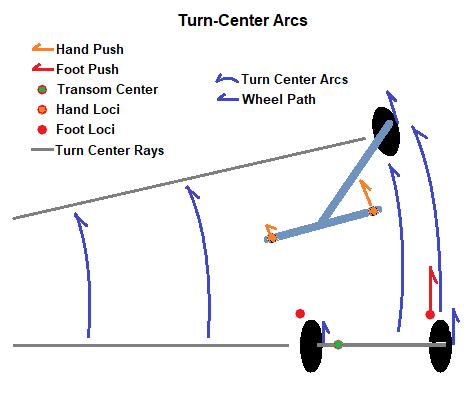
in a Cambered Left Turn.
Turn-Center Arc: A Trikke is physically constrained to move along a Turn-Center Arc as illustrated in Figure 10. Two gray rays parallel to the wheel axles meet at the Turn-Center off the left edge of the figure trying not to be noticed. Any arch equidistant from the Turn-Center is a Turn-Center Arc. When the Trikke turns right, the Turn-Center moves off the right edge of the figure. Again, your Trikke cannot move on any other path, it is stuck on an arc like an antique record player needle in a vinyl record. Like walking a box across the room.
The dance of riding a Trikke coordinates your generation of angular momentum along a Turn-Center Arc with steering and cambering the Trikke. A turning Trikke given enough angular momentum accelerates; all twisting and force channeled into a Turn-Center Arc builds its angular momentum - this turns the "magic lever".
Motion along any other path, does not contribute to Trikke movement; it only increases tread friction and frustration. All driving motion follows a Turn-Center Arc. Co-rotation - you and your dance partner; this makes the Trikke magic.
Notice that Turn-Center Arcs lie in the plane of the pavement. Vertical motion does not lie in that plane. Except for cambering, explained below, consider it useless. Generate motions parallel to the ground at all times.
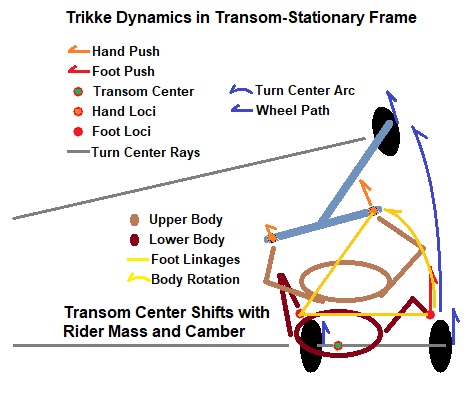
Effort in Orange and Red
Not only does the "Box-Trot" work to move your Trikke, the Trikke is designed to amplify it. After basic body position, cambering and pushing help leverage the angular momentum you create illustrated from a top view in Figure 11. Your feet cannot separate until you camber the steering column. Camber it by lowering your inside hand or raising your outside hand or both, slanting the top of the steering column toward the inside of the turn as in the figure. Cambering adds no motion along any Turn-Center Arc and does not change steering. It allows your feet to separate just enough to straighten the outside knee a little. The outside foot does not move on the deck, but it feels a push across the top of the deck (red arrow); never into it - that is wasted vertical motion. Avoid pushing into it by lifting your heel slightly.
Push the outside foot (red arrow) and hand (orange arrow) much more forward than their inside counterparts. The inside foot supports your upright body and does not push at all. Use the inside hand to keep its side of the handlebar stationary - something like rowing the handlebar, where your inside hand is an oar lock. Synergism again follows:
- It forces your body to twist slightly into the outside arm push. When you are used to it, especially if you can feel the power, amp up the body twist.
- It helps you throw a harder outside foot-push as your hips twist with it.
- Your body moves backward slightly adding to the push into the arch path.
Notice that cambering with your body upright also produces three synergistic benefits:
- Allows your feet to separate giving your outside leg the opportunity to push into a Turn-Center Arc.
- Cambers all of the wheels adding traction and stability in the turn.
- Shifts your weight toward the inside wheel contact point with the ground, decreasing friction on the outside wheel - because you are pushing across the outside deck, not down on it.
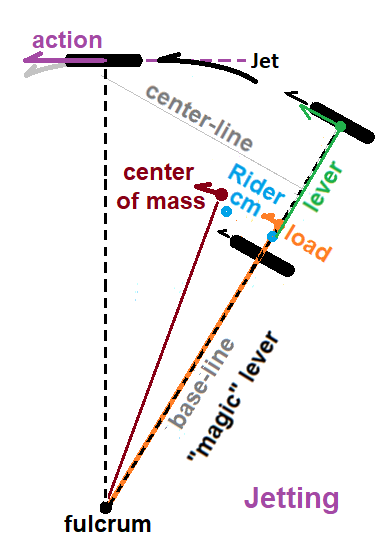
Note that the pivot in the Box-Trot is a foot or equally, a corner of the box. Co-rotation of the box and body utilizes the same pivot axis. On a Trikke these are separated. The body rotates about its own center of mass, while the Trikke rotates about its Turn Center. Physically, the body rotation translates to the Turn Center via the Parallel Axis Theorem. Thus we can correctly think that the body rotation pushes the green lever in Figure 12 with the force of the green arrow. The green lever is part of the rear-axle turn-lever which is functionally equal to Figure 3 the Magic Lever because they turn as a rigid unit. So body rotation feeds into a push of the Magic Lever; jetting.
Your Trikke is your new dance partner. You will learn to feel the dance from one side to the other; smoothly and quickly. In your mind's eye, see yourself gliding through this dance - videos of others riding a Trikke may help. However, like trying to learn a language from songs, the cues in those videos are likely too subtle or easily misinterpreted. Does the Trikke turn the rider or vice-versa? The rider must always turn the Trikke, unless coasting.
For example, it may feel like you or look like the person in the video is pushing hard on the outside foot and "unloading" the inside foot. That does happen through rotation you can't see or feel easily. However, the center of mass remains closer to the inside wheel and not where the push is directed over the deck - unless the inside arm of the Trikke is broken. A good rider keeps his head stationary, horizontally and vertically with respect to the trail while slinging the Trikke under his swaying body. The diagram in Figure 14 is a simple representation and a bit exaggerated for clarity.
Physics, math and computer based analysis guided by riding experience provided the foregoing explanations of the magic bottled up in a Trikke. Let's take advantage of them to ride a Trikke with grace and conservation of effort.
Starting Out
The easiest way to begin a ride is to grab the handlebars, push the Trikke forward a few steps and hop on. You're rolling! Don't need a simulation for that. A carving purist however, grabs the handlebars, steps up onto the decks, turns the guide-wheel about 80-degrees to a favorite side and torques into a turn at a practiced jet-speed. This won't be easy to accomplish at first and on rough ground it will always be difficult. It requires balance, cultivated body twist and foot thrust. If it's important to learn this right away, start pointed slightly down hill. Otherwise, wait until you've mastered carving and it will come naturally.
Start with your feet touching the back of the decks and your weight close to the inside turn wheel. Keep the steering column light. You want the turn-center and your center of mass to be close to that inside wheel so you can spin around it. This also aligns your body axis better with the minimal rotation inertial axis so the turn can be quick. There are a few body movements to coordinate simultaneously. Perform these movements illustrated in Figure 14 together, as quickly as you dare.
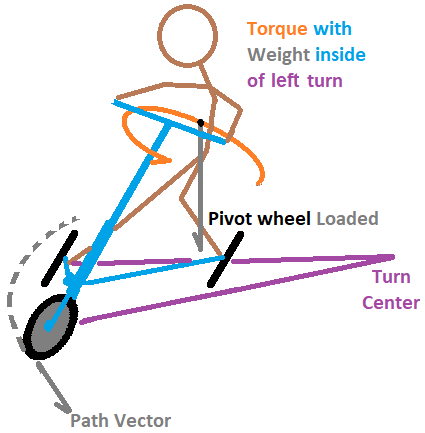
outside foot and hands around
- Pick a side to turn towards.
- Balance closer to that inside wheel with feet at the back of the decks by carefully leaning backward and toward the inside of the turn.
- Push the outside deck forward with your toes, heel off the deck.
- Twist into the chosen side. Your body rotates starting from the inside hip as a unit around your inside foot, upper body leading slightly. It feels like you are hooking your outside foot around toward the front. This is the most difficult part.
- With hands on the handlebars, push both forward, but more on the handlebar outside the turn.
- The core of your body is pushed backward slightly, but don't lean excessively to the side or hunch over; squat a little if needed. Let your feet and legs deal with the slanting tip of the decks.
- Don't camber yet, you might not have enough speed to remain stable.
- Quickly turn the handlebars to one side about 80-degrees.
When pushing the deck with your foot, do so without pushing down into the deck; keep the push across the top as much as you can. This goes for pushing the steering column as well. Push forward, not down. Pushing down diverts your energy into deforming the wheels' contact patches generating heat and friction instead of speed. It may help to raise the handlebars higher; standing straighter levels the foot and hand pushes. This is all part of keeping the guide-wheel light.
Gaining Speed
Once your Trikke is rolling, the best way to gain speed is also the fastest way. When I first rode, I tried what felt like rowing at the bow of a boat to ramp up the speed. I understood rowing. My arms felt like they were going to fall off, but I was riding my Trikke! The tires wore out in a couple of months. Carving properly is so much easier and much less wear on the tires. Carving requires balance, cultivated body twist, outside hand and foot thrust; i.e., jetting. Carving will likely take a few weeks to learn well if you work at it every day.
Starting from a slow roll, the goal for the first couple of cycles is to gain momentum. At this slow speed, carving means keeping the turn-center and your center of mass close to the inside trailing wheel. As the Trikke begins to build momentum, you can move your feet forward toward the front of the decks if that helps. It may be easier to control your center of mass by leaning back and squatting a little. Standing too far back limits you to lean forward, which misaligns with the minimal angular rotation axis. Use similar body movements as in starting out, but begin to camber. These actions are performed together:
- Immediately after the previous turn, turn in the other direction.
- Balance close to the inside wheel by carefully leaning backward and shifting toward the inside of the turn.
- Push the outside deck forward with your toes, heel off the deck.
- Twist away from the turn, but shift into it a little.
- With hands on the handlebars, push both forward, but more on the handlebar outside the turn, keeping the guide-wheel light.
- The core of your body is pushed backward slightly, but don't lean excessively to the side or hunch over; squat a little if needed. Let your feet and legs deal with the slanting tip of the decks.
- Camber into the turn as deeply as you can without making steering difficult.
- Turn the handlebars, to the other side about 70-degrees, decreasing the angle as you speed up over a couple of cycles.
Repeat the above movements increasing the cadence up to your normal carving drive-cycle; mine is about 1.5 seconds. Reduce the turn-angle and deepen cambering as you get closer to top speed. At top speed, turns will be very slight, camber and cadence optimized. Jetting feeds directly into the almost straight guide-wheel path. The "magic" lever works near its top efficiency with the turn-center some distance to the side; it lines up with your trailing wheels. Jetting practically straight with almost all camber really feels like magic because it seems like pushing yourself forward. And we all know that kind of perpetual motion can't happen.
Top Speed
As you reach terminal velocity, turns are very slight and cambering is maximized. If you were leaning your body side-to-side, that will decrease; naturally limited by less wide turns. It is only natural at this point to be riding practically vertical, leaning back and squatting slightly. Because you are leaning back, steering is easy and the guide-wheel load is light, which reduces its friction. Cambering feels more like thrusting the handlebars side-to-side away from you. Some might call it punching. The outside foot still pushes forward into the guide-wheel path, but feels like an extension of your body twist. Because you are vertical and the Trikke cambers under you, the total center of mass hovers near the center-line. This increases the geometric turn-radius ratio so that jetting is more efficient and helps the trailing wheels share friction more evenly. When most of the load is on one wheel, its friction can be much greater than the total friction when the load is equally shared.
Understand the [physics] and the technique will come. Rely only on technique and it will eventually fail.
Over Hill and Dale and other assorted topics
- Carving against resistance can be similar to starting from a stop or more like carving up inclines or against mild wind. Remember pushing and quick, wide turns "step" the Trikke forward. When you can't keep momentum flowing, step your Trikke. However, if your arms can take it or build up to it, stepping will always help your speed. Even so, the benefit decreases with higher speed. It is better to increase jetting capacity.
- Hills and wind affect Trikke speed similarly; they are resistance. They can be conquered with effort. A Trikke can climb a 6% grade and overcome a 20 mph wind from a stop. Place your center of mass as close to the inside wheel as possible, twist and push into the turned guide-wheel path. Keep the guide-wheel light, though there is a trade-off with leaning back too far and popping a wheelie. Once there is some momentum, quick, wide carving aligned with the minimal angular inertia axis is easiest. However, if you can jet well, try smaller turns with powerful jets keeping the guide-wheel light. If you have good enough momentum and the rise is only a couple hundred feet long, strong carving should suffice. If the surface is rough or wind too strong, just walk or run with your Trikke and save your wheels.
- Flying down hill? Keep carving as you would at terminal velocity. You will go even faster - at a higher terminal velocity! The jet-speed you generate is local. It builds your global guide-wheel speed, even when the Trikke is traveling faster than you can make it go on level ground. It may not seem like your efforts speed anything up because your jet-speed is a small fraction of your global speed. However, small contributions over many quick cycles add up.
Surfaces... Jetting directs your efforts into the guide-wheel path, which is ideal for traversing lose debris or water pockets strewn across a path. However, it's more likely a wheel may slip to the outside of a turn. When in doubt of your ability to judge the surface or your abilities crossing it there's always the option of coasting in a straight line or hopping off and walking around the hazard.
- Reduce the turning angle.
- Reduce cambering.
- Use more Direct-Push to "step" across, but only turn at a shallow angle.
- Keep the guide-wheel light; running over rubble with a "heavy" front wheel increases friction unnecessarily. Keep this friction for the really heavy trailing wheels, which can scatter, smash or deform the particles to reduce their impeding effects.
- If you frequently encounter debris, in addition to wearing a helmet, consider elbow and knee pads. Avoid backpacks in case you end up rolling as I have (and thanked God for self-defense training).
Tire tread and pressure are key to minimizing surface contact. On a relatively smooth concrete trail, smooth or "slick" tires pumped up to maximum pressure reduce carving effort. Tread with bumps behaves just like roads with bumps; they are self-inflicted bumps on an otherwise smooth road. They follow you around wherever you go. However, bumps may help scatter moisture and grip better after a rain. They are great for training and gaining body strength. Eventually, they become slicks.
- How does jetting with both feet work? To start this gait on flat ground, decrease cycle time while alternate hemijetting. That decreases the length of each hemijet until they almost naturally blur together. Make sure to squat and lean back by pulling on the handlebars. This aligns your twisting with the minimal inertia axis for faster turning and lightens the guide-wheel. The total (TRS) center of mass is typically slightly in front of you. Imagine trying to swing it in the same direction as the guide-wheel path; as if you are a twisting paddle and it is a ping-pong ball rolling on its surface. It is more strenuous to maintain than normal carving because it feels like there is no break in the action. Just one continuous, smooth chasing motion. I have not found this gait to be faster than my normal alternating, assisted hemijetting (enhanced Box-Trot), but it is a fun variation.
Temperature affects terminal velocity. Anytime a wet lubricant mediates the motion between two surfaces, heat normally affects its performance; it's more viscous on a cold day. On a cold day, try as I might, my tracker records show that I cannot propel my Trikke to the speeds I achieve on a warm day. They can be off by a couple miles per hour under otherwise equal conditions. "Equal" conditions are hard to maintain: I wear more layers, socks and gloves in near freezing temperatures. Perhaps my mental state is affected. Maybe I'm just slower in colder weather!
What is the "Sweet Spot"?
A "sweet spot" in a physical process is technically a minimum, maximum, inflection or resonance of some characteristic or behavioral quantity of the process. When [calibrating] the Trikke [simulation] the measured friction profile had a minimum at about 4.8 mph, about 2.14 m/s in Figure 15. That means the Trikke "wants to" or is most easily coxed into operating at or near that speed.
Friction actually reduces when the Trikke speeds up from standing still as if it wants to go faster. Accelerating past the minimum friction speed, makes the friction increase. Past about twice the minimum (green zone), friction becomes much more difficult to overcome (red zone). 12 mph (5.3 m/s) is about my terminal velocity on a flat, smooth road with no wind on a warm day. At terminal velocity, the rider's jet-speed matches the speed lost to all forms of friction. Note, the profile was measured for the one Trikke I had at the time. Yours will likely have the same form, but could be shifted or widened.
Another sweet spot is the presumed resonance I feel when jetting with both feet. By cambering and pulling on the handlebars, I provide the restoring force necessary to create the resonance. I can tune the sweet spot to whatever speed I like. Though it doesn't take as much energy as rapidly jetting with one foot at a time, it takes considerably more effort to maintain than normal carving. This may be a sweet spot in a high energy state, like "passing gear" in a car.
In online videos, I don't think "sweet spot" means either of these. I think it simply means a rider has figured out how to carve. The simulation shows that for my Trikke, I have to provide about 2 m/s
What is "Punching"?
Videos mention punching in a few different contexts and seem to explain it as a vertical force on the end of the inside handlebar or a quick thrust forward on both handlebars. The vertical force is quick cambering; forcefully pulling or pushing the guide-wheel across the forward path. By itself, the simulation shows it accomplishes nothing. However, it is part of good technique for reaching top speed with strong carving. Both hands punching forward is a quick, direct push. That is effective at stepping the Trikke forward in a turn, or after one if followed by a turn before resetting position. When directed around with a turn, this punch can also recruit the castering levers to step a little more. So, punch either way; it can only help.
What is "Leaning"?
Like "sweet spot", it is difficult to know what people mean by "lean".
- There is leaning backward to align the rider's body axis with the Trikke's minimal angular inertia axis to make steering and cambering easier. I recommend doing that once you are comfortable with the limits of Trikke stability.
- Forward lean - probably not a great idea. It places too much weight on the guide-wheel, which increases its portion of the friction load, especially on bumps and small rises. It's best to keep the weight on the slower trailing wheels. The slower the wheel, the less friction after 5 mph is achieved according to the friction profile in Figure 15.
- Sideways lean is consistent with trying to throw mass into a turn. That moves the system center of mass closer to the turn-center and actually reduces the amount of jet-speed transferred to the system center of mass via the turn-radius ratio. However, it keeps the rider's body axis aligned better with the Trikke's minimal angular inertia axis, so twisting produces more jet-speed. Which effect is greater, loss or gain of jet-speed? The simulation shows that since it is primarily a radial motion with respect to the turn-center, it dissipates in friction across the wheels and does not add to Trikke speed. My experience is that it doesn't generally help. I'm in an upright position for my fastest runs as described above.
- More recently, I noticed someone use this word to mean "Cambering"; tilting the steering column side-to-side. The simulation was pretty clear that cambering was not a major factor from a configuration point of view. The simulation does not cover jet generation; the kinesiology behind jet production. As indicated above, cambering can amplify the Box-Trot. So it is an important factor in jet generation allowing the rider to get the distance and angles necessary to plant feet in the right places and produce major muscle movements powerfully and comfortably. All things that were not simulated. This kind of lean can be very beneficial.
- Still not sure what the slogan, "Trust the Lean" means. Perhaps it's an appeal to trust the stability of the Trikke while cambering. Maybe. It took me a while to trust that stability and not be afraid of falling or slipping off the decks as they slant or of a wheel slipping. Learning to remain substantially erect between the three wheels helped immensely.
Saga of the Trikke Ballerina
Starting in the "prior art" patents to J. Gildo Beleski Jr. Cambering Vehicle and Mechanism. US Patent 6,220,612 B1. Dec 20, 2005, the idea that the Trikke-like vehicles worked somehow based on the Law of Conservation of Angular Momentum alluringly illustrated by a ballerina drawing her arms in was spun. The poorly explained association continued though each patent indicating to me that they had no idea about how it actually works or that since the appeal worked in the previous patents it was seen as necessary to obtain following patents. I suppose had they explained the "magic lever", it would not have been seen as so mysterious a mechanism as to deserve a patent.
Unfortunately, this myth has been propagated in Trikke literature, not just owner's manuals but training and "educational materials" targeting schools as well.
Why can't the Trikke Ballerina explain Trikke movement? An elegantly spinning ballerina does illustrate conservation of angular momentum and conservation of energy beautifully. But precisely because of that, she can never make a Trikke move! The problem is - she has constant energy. She spins faster as she pulls her arms inward, but in order to do that she cannot give away any energy; she has to keep it, conserve it. The Trikke needs energy to offset that lost to friction and to produce kinetic, forward motion. Energy the ballerina is not able to give to it.
Now, consider the ballerina alternating spinning directions, like a Trikke rider. To change directions, she cannot conserve energy because she must stop spinning at some point to change directions - her energy becomes zero! Suppose she manages to transfer that rotation to the Trikke, like a real rider. When she changes spin direction, to conserve the energy she gave away, she must take it back to spin up again - all of it! The Trikke ends up with no net energy from the ballerina.
A Trikke must gain energy from its rider, not conserve it. The rider is not a ballerina. The rider is more like a washing machine agitator motor accelerating to spin up the Trikke in alternating directions. Conservation of angular momentum is essential in constraining various aspects of the physics models representing Trikke locomotion, but it is not its principle of propulsion. Conservation can't explain that; Trikke propulsion is an open system. Like riding a bike, it will go faster if you work harder - up to external, terminal conditions. The principle of Trikke propulsion can be analyzed as alternating co-rotation from an angular viewpoint or pushes of an alternating "magic lever" from a linear one. Neither is a "conservative" process.
Experiment on Your Trikke
Fred Walsh, the National Trikke Trainer, showed me these exercises that follow. I practiced the first one every day before my regular rides for months afterward. It was really helpful. Only played the second one with Fred so far; and that was a bit one-sided. If you ever get a chance to take a lesson from Fred, do it. Even if you're a pro, you'll learn a few things.
Glossary
Camber, cambering Wheel tilt angle from vertical rotated around a wheel's forward path direction. Tilting a Trikke's steering column serving as the Camber-Lever to the left or right side creates a nearly equivalent vertical tilt in all three wheels. Foot-deck tops also angle synergistically to increase grip in a turn. For Trikkes, cambering generally refers to tilting the steering column from side-to-side coordinated with steering. Cambering enhances the effects of carving and castering.
Camber-Lever A second-class lever of the stem with fulcrum on the road and action from cambering that moves the yoke. It also interacts with the two levers involved in castering.
Camber thrust When a cambered wheel rotates, tread-particle elliptical trajectories are constrained to run straight when contacting the ground. This asymmetry causes a change in their momentum (a force) at right angles to the inside of the camber angle. This force and increasing change in camber pull the wheels into the turn avoiding dangerous wheel slips to the outside.
Carve, carving Most dictionaries don't define a sports sense of this word! [sportsdefinitions.com] does for skiing and skateboarding, basically a turn accomplished by leaning to the side and digging an edge or wheel into the path. In the case of "carving vehicles" or "CV"s, carving is a transfer of momentum from the rider to the vehicle synergistically constrained by ground forces on the wheels. The Trikke seems best engineered to claim this action as a primary form of locomotion. This motion mechanism provides action to the guide-wheel contact point around the Turn-Lever.
Caster, castering Most dictionaries don't define a sense of this word as a verb! Here, it is defined as a form of locomotion. To caster is to move by quickly turning a wheel with positive caster and positive trail back and forth to produce forward motion. The offset contact patch causes the vehicle frame head or yoke to move sideways a couple of inches pulling part of the vehicle's trailer mass into the turn. Impulse created by this motion in the direction of the drive wheel path first pulls, then pushes on the vehicle's stem assembly. It is the sideways rotation not the stem pull or push that creates a small jetting impulse. This appears to be the primary means of locomotion for the [EzyRoller], [Flicker], [PlasmaCar] and Wiggle Ride-On Car by Lil' Rider (no web presence) rider toys and one of the modes for a Trikke. Two levers mechanize this motion: the Yoke-Lever and the Trailer-Lever.
Caster angle - positive, negative The angle a steering column makes with the road. It is positive when the angle slopes up toward the back of a vehicle. It is negative otherwise.
Caster pull When the handlebars of a Trikke are turned and tilted quickly toward the center, the geometry of the steering mechanism and constraints on wheel motion, throws the yoke into the turn up to a few inches and pulls the Trikke's Extended-Stem and trailer a quarter inch or so closer together in a fraction of a second. Though the rotation is small, most of the weight of the Trikke and its rider get spun around the transom point. The pull generates no immediate jetting impulse. Steering resistance increases as caster pull builds local angular momentum.
Caster-push When the handlebars of a Trikke are turned and tilted quickly away from the center, the geometry of the steering mechanism and constraints on wheel motion, throws the yoke into the turn up to a few inches and pushes the Trikke's Extended-Stem and trailer a quarter inch or so apart in a fraction of a second. As the turn reaches its limit, the built up angular momentum transforms into a jetting impulse. The rider synergistically leverages this push for speed by synchronizing carving impulses with it.
Citizen scientist An individual who voluntarily contributes time, effort, and resources toward scientific research in collaboration with professional scientists or alone. These individuals don't necessarily have a formal science background. See What is citizen science? [Citizen Science].
Conservative force A force that can be expressed as the gradient of a potential. When this is possible, the work done by the force is not dependent on the path taken. A "round trip" by different paths of the same length requires the same amount of work. Gravity is a familiar conservative force.
Design Of Experiment (DoE) A type of designed experiment contrived to efficiently identify (screen for) the control factors that affect the process being studied the most (main effects). DoE can model and compare the effects of several factors against each other using Yates analysis. It attempts to optimize the differences in effects and minimize the number of trials needed to obtain them. When models are attained they have least-squared, multilinear properties subject to the confounding structure of the experimental factors. When not attained, the true process model is non-linear.
Direct-Push and Pull, slinging Intentional impulse created as the rider quickly moves his or her center of mass in the opposite direction of the handlebars. Lightening the load on the drive wheel and pushing the Trikke forward with arms and legs feels like "slinging" the Trikke forward. In order to retain the ground gained, this maneuver must be concurrent with or followed by steering, cambering or both. During the push or pull, the mechanism produces a jetting impulse; acceleration for the push, deceleration for the pull.
Dynamics Study involving variables related to the generation of an object's motion. Answers questions about how motion is produced.
Equation of motion (EOM) Application of conservation laws or principles like least action and balance of forces and torques to produce global kinematic and other equations for a system. For example, an approach due to Lagrange starts with energy conservation, equivalences between kinetic and potential to derive system velocities, then other kinematic quantities and sometimes Newton's force-based EOMs can be derived for the system. D'Alembert's principle allows various constraints on part velocities and positions to be incorporated in some EOMs. Other approaches create state-based generalized coordinates and use lie algebras to represent system functions. While many EOMs cannot be solved symbolically, they lend themselves to numerical solutions via computer simulation.
Extended-Stem The part of the TRS that is turned by the rider during castering. Composed of the stem, hands and wrists of the rider's body. Does not include body parts considered part of the trailer. In lever systems, one-third of a linkage between two moving parts can be shown to act as if stationary with respect to the closest attachment. So, one-third of a hand and arm acts as if stationary to the stem, another third to the trailer and a third acts with the arm's own momentum.
Extended-Trikke The part of the TRS that slings during carving. Composed of hands, wrists, feet, ankles and parts of the rider's body that sling with the Trikke. The part of the reduced rider's body producing the jetting and Direct-Push impulse is not part of the Extended-Trikke. In lever systems, one-third of a linkage between two moving parts can be shown to act as if stationary with respect to the closest attachment. So, one-third of a foot and lower leg acts as if stationary to the deck, another third to the rider and a third acts with the lower leg's own momentum.
GCF Global Coordinate Frame, "lab" or "observer's" frame may contain an infinite number of LCFs twisting, moving and even changing size everywhere. The Trikke-Rider System LCF is fairly well behaved inside the GCF; it has a common z-axis and doesn't change scale. Observers can see and measure the TRS as it moves and turns and can all obtain the same values. But they can't feel the accelerations of the Trikke like the rider.
Jetting, hemijet The angular component of carving. Beyond the "natural" body twist accompanying slinging, the rider extends the foot outside the turn by lowering his center of mass, leaning back and twisting the hips into the turn. This angular impulse adds angular momentum to the system via the Parallel Axis Theorem through rotation about the TRS center of mass. The word comes from an outdated skiing technique using both feet. Technically, this is "alternating hemijetting" since each foot independently executes half of a "jet" (a hemijet) in one drive-cycle. However, it is possible to jet with both feet.
Kinematics Study involving variables related to the classification and tracking of an object's movement. Answers questions about the form and characterization of motion, not how the motion is produced.
Lateral plane An imaginary plane that separates the front half of an object from its back half. Its normal is parallel to the front-to-back axis of the object.
LCF Local Coordinate Frame, inertial frame or just local frame is the rider's world where the Trikke and everything on it seems relatively stationary compared to the rest of the world. It is "inertial" because the Trikke and rider "feel" centripetal force in a turn and the accelerations of its movement. Yet the Trikke remains close to the rider. "Forward" is pretty much ahead. There is a wheel always under the left foot. Things are local and for the most part in their expected places. When the rider measures things relative to his locality in the GCF, they are usually different than the same things measure by observers in the GFC.
Magic Lever Any Turn-Lever passing through the front-wheel axle or transom used to think about or compute the jetting reactions of the Trikke. Really, any turn-lever will do as they all move as if embedded in a solid, but the two are the simplest to use in mathematical models.
MA, Mechanical Advantage A ratio, percentage or number expressing the force multiplier or efficiency of a lever system. For simple levers, it is the distance of the action to the fulcrum divided by the distance of the load to the fulcrum.
Nonholonomic system (also anholonomic) In physics and mathematics a nonholonomic system is a type of system that ends up in a different state depending on the "path" it takes. In the case of the Trikke, frictional forces and some geometric constraints restricting velocity but not position prevent the system from being represented by a conservative potential function. It is "non-integrable" and not likely to have a closed-form solution.
Normal A "normal" to a plane, is a ray starting at the plane which is at right angles to every ray in the plane that starts at the intersection point. Notice that the normal cannot lie in the plane. Its unit direction is also the "direction" or "orientation" of the plane.
Parallel Axis Theorem One of two theorems by this name. Translates a moment of inertia (moi) tied to a center of mass and rotation axis to an offset but parallel axis. If the moi is expressed as a tensor with no particular rotation axis, then it translates to an arbitrary point, usually on the object. The second namesake does the same for an angular momentum vector. There is also a theorem called the "Second Parallel Axis Theorem" which is simpler to apply in some contexts.
Reduced rider About fifteen percent of the rider's body mass acts as if it is a part of the Trikke. Hands and wrists move largely in unison with respect to the handlebars. Corresponding parts of the legs act as if part of the decks on the arms of the Trikke. This "reduces" the effective mass and distribution of the rider, while increasing that of the Extended-Trikke and Extended-Stem.
Sagittal plane An imaginary plane that separates the left half of an object from its right half. Its normal is parallel to the left-right axis of the object.
Steering column, stem The controlling mechanism surrounding the steering axis. Composed of the steering column, handlebars, rider's hands and parts of his forearms (about 1/3) as well as the yoke, front wheel, axle, bearings and attachments. It is considered part of the Extended-Trikke, but is the part of the TRS not included in the trailer. It is also a lever; the Camber-Lever.
Stride A body in motion has linear and rotational (or angular) velocity. Parts of the body have different velocities depending on their distance from the rotation center. Stride is the difference between a part's velocity and the linear velocity of the whole body at its mass center. Rotational velocity can be expressed as a linear velocity, v̅, at right angles to a radius pointing from the rotation center: (v̅ = ω̅ × r̅). When the rotation center is the turn-center of the TRS, and the radius ends at a wheel, the difference between v̅ and the TRS linear velocity is that wheel's stride.
Swath As a Trikke snakes its way along, the outer edges of the wheels trace a wide ribbon-like path down the road. At terminal velocity, if one connects the outer most edges of the wavy ribbon on both sides to get a long rectangle, the width of that outlined area is the "swath" covered by the Trikke. In simpler terms, it is the width of the path covered by the Trikke. If something is within the swath of the Trikke, there's a decent chance it will be hit depending on Trikke's trajectory.
Trailer The parts of a TRS that are rotated around the transom point and pulled or pushed slightly by the Trailer-Lever while castering. Consists of the parts of a Trikke other than the steering column, front drive wheel, handlebars, "handlebar-stationary" hands and parts of the forearm (about a third of it).
Trailer-Lever Caster action is applied at the yoke by the Yoke-Lever which rotates it and pulls or pushes it. The center of mass of the trailer is its load. Its fulcrum is on the Transom point. The Trailer-Lever is a second-class lever and push-rod.
Transom Used to indicate the points on the line between the rear wheel contact points with the ground or axles. A point along the transom instantaneously acts as the Trailer-Lever fulcrum. Its position is determined by the ratio of instantaneous friction at each wheel. Thus, when the load is nearer the left wheel, the transom point, is nearer the left wheel. For equal friction on both wheels, it is in the center of the line between contacts.
TRS Trikke-Rider System. Refers to the entirety of the physical system composed of the Trikke, all of its relevant parts and behaviors and the rider with all relevant parts and behaviors needed to complete the current investigation. Though the road, air and other parts of the environment are required to operate the system, they are not considered part of it.
Turn-Center Arc As a part of the Trikke turns around the turn-center it traces a circular arc or orbit at a constant radius until the steering angle is changed or a wheel slips. Note, each part has its own orbit, closer to or further from the turn-center. Those further out travel around at a faster speed because their orbits are larger. Consider also that parts of the rider turning with the Trikke have their own turn-center arcs concentric with the parts of the Trikke.
Turn-Center A Trikke orbits this stationary point at a constant radius until the steering angle is changed or a wheel slips. When steering straight, the turn-center is not defined and the turn-radius is considered infinite. Jetting makes use of the turn-ray as a second-class lever acting on the system center of mass.
Turn-Lever A turn-ray is the ray from the turn-center to a point on the Trikke. When the point is the guide-wheel contact point or on the transom, the ray becomes an important motive lever as all TRS motion must move the contact point around the turn-center.
Yoke Idealized point of intersection for the three structural tubes that characterize a Trikke. This genius articulation is fairly complex and precisely manufactured. It is the soul of the Trikke - if there is one. Constant motion from steering, cambering and road vibration are robustly endured, while keeping all the wheels aligned without toe-under or splaying.
Yoke-Lever Constitutes part of the castering mechanism set in motion by turning the stem. The rotation produces an action at the yoke, which is also the load. Its fulcrum is the guide-wheel contact patch. The movement of this yoke-load acts on the Trailer-Lever which rotates it and pulls or pushes it. The Yoke-Lever is a degenerate lever.
References
Patents
[Trikke Patent] J. Gildo Beleski Jr. Cambering Vehicle and Mechanism. US Patent 6,220,612 B1. Dec 20, 2005. Google Patents US6976687B2. Prior: 1999 US09434371 Active, 2000 US09708028 Active, 2002 US10331558 Active, 2004-06-25 US10876497 Expired - Fee Related, 2004-11-18 US20040227318A1 Application, 2005-12-20 US6976687B2 Grant.
Control Theory in Robotics
[RoboTrikke] Sachin Chitta, Peng Cheng, E. Frazzoli, V. Kumar. "RoboTrikke: A Novel Undulatory Locomotion System". In Proc. IEEE Int. Conf. Robotics and Automation, pages 1597-1602, Barcelona, Spain, April 2005. DOI: 10.1109/ROBOT.2005.1570342.
[Roller-Racer] P. S. Krishnaprasad and D. P. Tsakiris. "Oscillations, SE(2)-Snakes and Motion Control: A Study of the Roller Racer." Technical report, Center for Dynamics and Control of Smart Structures (CDCSS), University of Maryland, College Park, 1998.
[Roller-Walker] Gen Endo and Shigeo Hirose. "Study on Roller-Walker: Multimode steering control and self-contained locomotion". In Proc. IEEE Int. Conf. Robotics and Automation, pages 2808-2814, San Francisco, April 2000. DOI: 10.1109/ROBOT.2000.846453
Papers
[CarveABike] Sachin Chitta, V. Kumar. "Biking Without Pedaling". November 2006. Chitta, Sachin. “Biking Without Pedaling.” (2006).
[Air Force] Charles E. Clauser, et al., Air Force Systems Command, Wright-Patterson Air Force Base, Ohio. August 1969. Pub AD-710 622.
[Moment Of Inertia] Dr. J. B. Tatum. Chapter 2, 'Moment Of Inertia', section 2.8. University of Victoria. 2017. http://astrowww.phys.uvic.ca/~tatum/classmechs/class2.pdf, 2019.
[physics] Michael Lastufka, "Body-Powered Trikke Physics" June 2020.
[calibrated] Michael Lastufka, "Empirical 2nd Degree Friction Model Solution" June 2020.
[simulation] Michael Lastufka, "Dynamic Model of a Trikke T78 Air" June 2020.
[behaviors] Michael Lastufka, "Survey of Simulated Trikke Behaviors" June 2020.
[Quick Start] Michael Lastufka, "Trikke Magic: Start Carving" June 2022.
[co-rotation] Michael Lastufka, "Qualitative Body-Powered Trikke Physics" November 2022.
[George Box] Box, George E. P. (1976), "Science and Statistics" (PDF), Journal of the American Statistical Association, 71: 791–799, DOI: 10.1080/01621459.1976.10480949.
[Nonstandard Analysis] MathWorld: Nonstandard Analysishttp://mathworld.wolfram.com/NonstandardAnalysis.html. 2019.
[POV-Ray]http://www.povray.org/, 2019.
[sportsdefinitions.com] SportsDefinitions.com. http://www.sportsdefinitions.com/, 2019.
[EzyRoller] EzyRoller LLC. 22588 Scenic Loop Rd., San Antonio TX 78255 - USA. https://www.ezyroller.com/, 2019. Email: info@ezyroller.com
[Flicker] Yvolution USA Inc. 2200 Amapola Court, Suite: 201, Torrance, CA 90501 – USA. https://yvolution.com/, 2019. Email: support@yvolution.com
[PlasmaCar] PlaSmart Inc. 228-30 Colonnade Road, Nepean, Ontario K2E 7J6 - Canada. https://plasmarttoys.com/, 2019. Customer Service 1-877-289-0730 Ext. 214
[Citizen Science] SciStarter. https://scistarter.com/citizenscience.html, What is citizen science?, 2019. Email: info@scistarter.com